Synchrotron radiation
1. What is SR?
A charged particle traveling in the external magnetic field is accelerated
under the effect of the Lorentz force and therefore radiates. In 1944 Soviet
physicists Ivanenko and Pomeranchuk established a theory of this radiation.
It was experimentally observed by American scientists Blewett and Haber (1947)
as light from electrons accelerated in a synchrotron and therefore was given
the name synchrotron radiation (SR).
Beginning in the 1960s, synchrotron radiation found wide use in various
fields of science and technology. At that very time synchrotron radiation
investigations were also started in Russia. They were carried out at the synchrotron
S-60 by scientists of FIAN (Physics Institute of the USSR Academy of Sciences)
and Moscow State University and at the VEPP storage rings in the Institute
of Nuclear Physics in Novosibirsk.
In the late 1970s and early 1980s special electron storage facilities built
exclusively for SR activities were put into operation one after another. This
"synchrotron boom" extended to entire branches of science and technology,
such as physics, molecular biology, chemistry, medicine, electronic industry,
and ecology.
The first theoretical and experimental researches of SR were started at JINR
about 25 years ago in connection with formation of relativistic electron ring
in the collective accelerator of heavy ions.
Investigations with SR are being rapidly developed in the world. There are
over 100 SR sources in operation or under construction in the World. Russia
has only two SR centres at the Budker Institute of Nuclear Physics (Novosibirsk)
and at the Kurchatov Institute (Moscow).
SR is produced actually in the circular parts of ring-shaped accelerators
and accumulator rings of electrons. Modern SR sources are equipped with special
magnetic elements (so called insertion devices) - wigglers and undulators
with the aim to produce SR up to 1000 times more intense than that produced
at an ordinary bending magnet of a ring accelerator.
SR of ring accelerators has a very narrow radiation beam and wide energetic
spectrum with wavelength ranges from 0.1 mm to 0.01 nm in dependence on electron
energy and strength of magnetic field in the accelerator structure.
2. Free Electron Laser (FEL)
FEL is a new type source of coherent radiation produced by a high-intensity
electron beam while passing through the undulator placed between cavity mirrors.
Over the last decades SR has turned into a most powerful research tool applied
in many different fields of science: in physics, radiation chemistry and photochemistry,
molecular biology, in material sciences, medical diagnostics and other fields
of science.

FEL generates tunable, coherent, high power radiation, currently tuning
wavelengths from mm to visible and potentially from UV to X-ray. It can have
a quality of optical properties of conventional lasers such as high spatial
coherence and a near diffraction limit of beam radiation.
The past decade has seen considerable advance in linear accelerator technology,
FEL physics and technology, which has opened up possibilities of creating
reliable and relatively inexpensive special infrared and ultraviolet FEL radiation
centres (FELIX in the Netherlands, CLIO in France, FELI in Japan, FEL centres
in some leading US universities, like Stanford, Vanderbilt, Duke). In the
near future it is planned to put into operation short-wave FEL in the Germany
(TTF FEL, wavelength 6-200 nm) and in the Argonne National Laboratory,USA
(wavelength below 150 nm).
3. What can SR do?
Among numerous areas where SR is widely used now, five should be particularly
mentioned. They are biology, medicine, micromechanics,
ecology, microelectronics and materials science.
Modern intense SR sources allow breakthroughs over several directions in biology
and medicine, including not only laboratory research but also practical
applications.
Biology
Historically, the first of these directions became X-ray
structural analysis of proteins, the pioneering investigations carried
out by a group of researchers from the Institute of Atomic Energy (now the
Russian Scientific Centre-Kurchatov Institute) and the Institute of Theoretical
and Experimental Biology (Pushchino) at the VEPP-3 storage ring in the Institute
of Nuclear Physics (Novosibirsk) as early as the 1970s. Now these methods
largely govern development of genetics, gene engineering,
and biotechnologies.
Apart from applications in this area, synchrotron radiation is planned to
be used for a wide range of investigations: study of such biological objects
as muscular fibres, including dynamics of structural recombination of living
objects (X-ray "cinema"); protein crystallography and investigation
of the structure of organic molecules; dynamics of proteins, the structure
of active centers of proteins and various biocatalysts; spectroscopy of biopolymers,
structure of biological polymeric films; elemental X?ray fluorescence analysis
of various biological objects, e.g. trace analysis of blood; medical and biological
fast and highly sensitive roentgenoscopy; microsurgery and phototherapy.
Medicine
The first to be mentioned out of the most promising medical applications of
SR is angiography, prompt roentgenoscopy of a
patient's blood vessels.
X-ray diagnosis with the use of synchrotron radiation
and new effective detectors, e.g. storing screens, allows a substantial decrease
in the radiation load to a patient.
Another equally important application of SR is elemental analysis of medical
samples, i.e. non-destructive testing and certification
of medicines sensitive to small impurities (including harmful ones)
indistinguishable by other methods.
Micromechanics
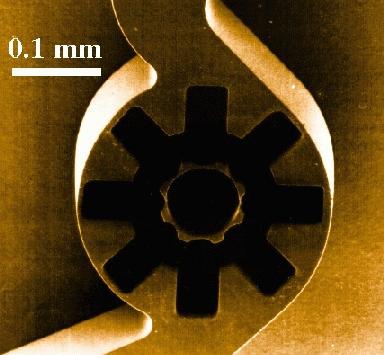
An intense SR beams can be used as a "microtool" for cutting materials
to dimensions and manufacturing various microscopic items, mechanisms, and
devices. This is the so-called LIGA process being actively developed now.
Typical dimensions of items produced with this technology is in order of a
few micrometres, which immediately points to medicine as the primary area
of their application through life-supporting mechanical devices (e.g. pumps
in the blood-vascular system). Now at the laboratory development stage, devices
like this will extensively progress in the coming decade.
Ecology
Currently developed highly sensitive SR-based methods of elemental (X-ray
fluorescence) analysis allow a qualitatively new level of environmental
control and also solve the problem of accurately measuring concentrations
of small admixtures of all elements of the periodical table. With SR, it is
possible to detect concentrations of elements at a level
of 108 of the admixture atom per atom. These methods make it possible
to analyze, for example, the composition of aerosols, precipitation, and admixtures
to water for ecological monitoring purposes.
Obviously, biological, medical, and ecological applications
of SR are directly related to human health.
Microelectronics
SR-based X-ray lithography, a process of depositing conducting coating onto
a crystal surface, allows the size of present-day electronic circuit elements
to be reduced by one-two orders of magnitude and thus the computer speed to
be increased. In the near future the potentialities of present submicrometre
technologies will be exhausted and transition to the nanometer level ("nanotechnologies")
will occur. Now the leading electronic companies of the world (IBM
and others) are involved in this kind of research and development activities.
Metrology
Synchrotron radiation is an ideal photometric standard
for the vacuum-ultraviolet (VUV) and X-ray radiation. It is good to provide
a photometric channel, for example, for absolute calibration
of electromagnetic VUV and X-ray radiation detectors. This channel can be
used for absolute calibration of dosimeters, detectors employed in various
fields of science and technology, including space research.
The above-mentioned applications of SR govern current
development of high technologies.
Materials science
Now SR-based methods of X-ray structural and elemental analysis are widely
used to develop new materials. Sensitivity and speed of the SR-based analysis
allows development of new materials to be raised
to a level unattainable with traditional methods.
SR is also used to develop new efficient luminescent detectors, in particular
scintillators.

Geology
SR methods are invaluable for elemental analysis of various ores and minerals.
They allow investigating the structure of submicron-thick surface layers of
materials, taking microtomograms with a resolution of a few micrometres, measuring
concentration of elements in liquid inclusions in minerals, in powders, etc.
The applications of SR listed in the previous section
are the most important and promising first of all in practical areas. Yet,
applications of SR in fundamental research are equally important.
Condensed matter physics
Condensed matter physics, including crystallography, is an area where SR-based
research yields fundamentally new results. SR is used in investigations of
the electronic structure of solids, high-energy excitation of luminescent
crystals, the structure of clusters and thin films, phase transitions under
superhigh pressure and in X-ray microscopy. The modern method for analysis
of the atomic structure of objects, EXAFS spectroscopy,
is being successfully developed with the use of SR.
Highenergy SR can be effectively used for high-resolution photoelectron spectroscopy
to analyse the surface of a solid and to investigate its phonon structure.
Nuclear physics
Generation of strictly directional beams in the SR flux, so-called Mossbauer
quanta, extends experimental capabilities of nuclear-physics investigations
(collective nuclear excitations, analysis of the magnetic structure of a sample,
etc.).
Astrophysics, space research
Elemental analysis of superhigh resolution is necessary to investigate samples
brought to the Earth by space vehicles or taken from meteor showers.
Some space investigations require absolute calibration of electromagnetic
radiation detectors, which is best achieved with SR sources.